The future of pale cyst nematode control
Potato cultivation in Idaho had its start, on a very small scale, in 1836. Farmers soon discovered that the state’s climate and soil yielded harvests large enough not only to meet their own local needs, but also to meet the growing demand for the tubers in markets elsewhere in the country.
Potato production began to make an especially significant contribution to Idaho’s economy after the introduction of Luther Burbank’s namesake variety in 1872. This light-skinned potato was subsequently replaced by the Russet Burbank cultivar that is so important today. However, as this commercial industry has grown, so too have never-before-seen threats to this success story like potato cyst nematodes (PCN).
The first example of this kind in the U.S. was the Golden Nematode (GN), Globodera rostochiensis, detected in 1941. It is thought to have been brought there years earlier in infected soil caked onto WWI military equipment parked in a farmer’s field on Long Island in New York.
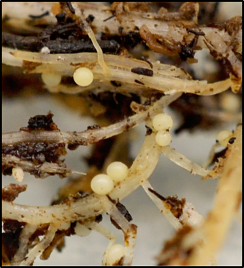
In 2006, a second infestation, not of G. rostochiensis, but of a related species, PCN (Globodera pallida), was detected in two counties in southern Idaho. Stringent quarantine procedures to limit the spread of PCN were quickly put in place and the infested fields were fumigated to eradicate eggs that were present. However, these pathogens encase their eggs within cysts derived from the bodies of their mothers (Figure 1). These shells can protect the eggs up to 30 years.
Complete eradication of the eggs in these reservoirs has proven to be a lengthy operation — it reportedly took 24 years to eradicate PCN from a 15-hectare region of Western Australia. More recent efforts carried out in Idaho have proceeded much more quickly, with many fields already returned to potato production with robust soil surveys until final deregulation. Nevertheless, some infested fields remain quarantined after 14 years.
Researchers continue to look for more rapidly implementable and longer-lasting ways to contain these parasites. Despite the improved tools that are now available through the use of molecular biology, plant breeding today differs very little from plant breeding performed 50 years ago.
Crossing two potato varieties still blends all traits of each parent, bad as well as good, into a single hybrid. It then takes many generations of additional crosses to reproduce a line with the desirable traits of Russet Burbank. Moreover, this tried and true method remains dependent on finding effective sources of PCN resistance in the small number of species that can be bred with domestic potatoes. A majority of the scientists surveyed in 2013 reported that no one could guarantee that these limited resources are able to offer a long-term solution to the problem.
In the end, the same investigators stated that breeders would eventually have to exploit the resistance traits of unrelated and incompatible species that can only be transferred into potatoes using the tools of genetic engineering.
A new possibility
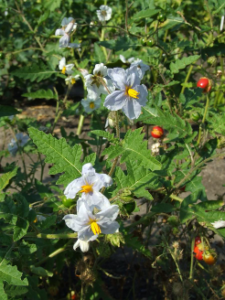
One such resource for potentially novel defense genes is a distant relative of potatoes and tomatoes called Solanum sisymbriifolium, and more commonly, litchi tomato (LT). This plant (Figure 2 and 3) has been studied off and on since 2000 for its ability to act as a trap crop, which is a plant that stimulates cyst nematode eggs to hatch and to invade its roots, but then poisons or starves the parasites. It effectively terminates whole generations of nematodes that have been lying in-wait for the next crop of potatoes and does so without the use of chemical pesticides.
Planting it in contaminated fields has been shown to dramatically reduce the number of viable PCN eggs in soil. Repeating these plantings in successive years can virtually eliminate all Globodera eggs, as well as those of several other nematodes. It stands to reason that if we could determine how litchi tomato protects itself against PCN and several other nematodes, we might be able to introduce the appropriate genes into potatoes to allow that plant to defend itself and thus eliminate the need for an independent trap crop that has no economic value.
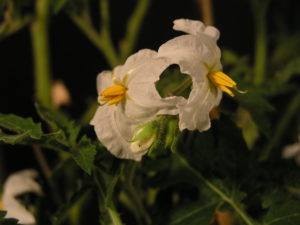
Over the last three decades, researchers have learned quite a lot about how to use genetic engineering to create disease-resistant plants. One of the earliest efforts to improve a plant’s natural resistance to disease was undertaken more than 25 years ago when a devastating plague caused by papaya ringspot virus appeared in Hawaii. For five years, it spread from plantation to plantation reducing the papaya harvest by 40%. Using technical approaches that had already proven successful against other viruses under laboratory conditions, the ringspot virus genome was isolated, sequenced, and then used to create novel genes that interfered with the virus’ ability to reproduce. Additional improvements led to the creation of fully immune transgenic lines of papaya that are still in use today.
We are not at this point yet in the development of nematode-resistant plants. For one thing, nematodes lead far more complicated lives than viruses, and we know far less about Globodera’s 16,000-plus genes than about papaya ringspot virus’ nine genes. Efforts are underway to correct this, but we simply do not yet know the best way to kill Globodera or inhibit its reproduction.
Adding knowledge
The first step toward filling in the many gaps in our knowledge were taken in 2020, when scientists at the University of Idaho published the world’s first database cataloging some of the changes in gene expression that occur when nematode-resistant LTs are infected with G. pallida. Previous work from the same group identified 41,189 genes expressed in different parts (including roots, leaves, and other organs) of healthy LTs. In addition, this earlier work revealed the complex history that this plant has experienced during its evolution. In particular, this study showed that the litchi tomato genome is quite a bit larger than the largest potato genome.
Large genomes like this are not uncommon in the plant world. It often happens that accidents of inheritance cause an individual plant to retain two copies of its own genome, creating what are called polyploids. Such individuals are often more vigorous and better able to outcompete plants with only one set of their genes. Moreover, genome duplications provide raw material for the refining processes of natural selection. One gene copy can retain its original function while the duplicate can be reassigned to perform a more specialized role in the biology of the plant. Thus, while potato and LT may have started with similar genes, the ancient genome duplication may have allowed LT to broaden its defenses against dangerous pathogens.
Having revealed most of the genes expressed in healthy LT, the UI researchers next focused on determining how the genes expressed in roots changed expression after Globodera pallida infections. During this focused survey, they found additional LT genes bringing the total count to 50,625. Of these, more than 277 genes showed significant changes in expression after nematode infection. More than one in five of those genes were unlike anything that could be recognized in the genome sequences of other plants, while as many as 10% more resembled genes that have been found elsewhere, but never investigated.
In spite of the large number of genetic novelties among the 277 potential nematode-defense genes, an analysis of those genes that could be assigned functions presents a picture of a multilayered defense against parasitic nematodes. Plants do not have an adaptable immune system like mammals that generates antibodies against individual pathogens. However, plants do share an independent kind of defense with people called the “innate immune response.”
In plants, this response is triggered when chemicals or proteins characteristically produced by different pathogens are detected by a specialized kind of host protein called a “receptor.” Plants have many different kinds of receptors, each acting as a guardian against a molecule associated with one or more kinds of pathogens; the UI team found at least six nematode-induced proteins of this kind.
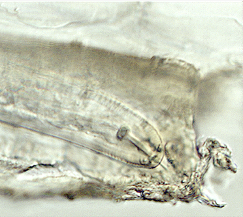
When these guardian proteins detect the pathogen-associated chemical signals, several different types of defenses are activated. Surprisingly, one of the most effective defenses a single plant cell can perform is to commit suicide through a process (also carried out when human cells are infected by our pathogens) called programmed cell death. This kills the infected cell, but in dying, it can also kill the invader before the invader proliferates and spreads to other cells. A surprising number of the 277 LT genes that increase in expression after PCN infection appear to be responsible for programmed cell death, and careful microscopic studies of infected LT roots has revealed nematodes completely encased in dead plant cells (Figure 4).
Equivalents of these genes are not induced in the roots of sensitive plants, like potatoes, which apparently either do not recognize the invader or lack a means of responding to it.
There are many reasons why a plant gene would change expression during nematode infection. While some might be part of the plant’s defense, others might become activated as the parasite tries (unsuccessfully) to reshape litchi tomato root cells into structures that would provide nutrients for the nematode. Other genes may be turned on or off as the plant attempts to preserve the structure of its root cells, but these are not the kind of genes likely to be directly responsible for killing the pathogen.
To be clear, just looking at DNA sequences cannot tell us which of these genes is changing its operation for the benefit of the cyst nematode, and which is changing for the benefit of the plant. To make those kinds of distinctions so that the defensive genes could be used to engineer truly resistant potatoes, researchers will have to study each candidate gene in isolation from the rest.
This will require putting each gene into potatoes, testing whether the resulting transgenic plants are more resistant to Globodera, and finally combining multiple genes in a single cultivar to re-create the litchi tomato defense system. This kind of analysis is already underway in laboratories in Idaho, New York and elsewhere, to generate crops that are as resistant to PCN as LT.
Work will also continue in order to identify potato cultivars with effective nematode resistance that could be bred into premier genetic backgrounds like Russet Burbank. But if these donors are not found, or if Globodera acquires mutations that break that resistance, growers need a “Plan C,” like genetic engineering to fall back on.
Traditional breeding, the development of new nematicides, and genetic engineering each have their own limitations. Just as healthcare services need to anticipate and prepare flu vaccines before epidemics begin, nematode resistant potatoes need to be developed either through classical breeding or genetic engineering before crop failure.
— Allan Caplan, Fangming Xiao, Joseph Kuhl and Louise-Marie Dandurand are professors and researchers at the University of Idaho. They contributed this article on behalf of the Potato Association of America.